Making a miniature heart - miniPUMP.
- pnegatina
- Apr 28, 2022
- 4 min read
Biomimetic on-chip tissue models serve as a powerful tool for studying human physiology and developing therapeutics; however, their modeling power is hindered by our inability to develop highly ordered functional structures in small length scales. Here, we demonstrate how high-precision fabrication can enable scaled-down modeling of organ-level cardiac mechanical function. We use two-photon direct laser writing (TPDLW) to fabricate a nanoscale-resolution metamaterial scaffold with fine-tuned mechanical properties to support the formation and cyclic contraction of a miniaturized, induced pluripotent stem cell–derived ventricular chamber. Furthermore, we fabricate microfluidic valves with extreme sensitivity to rectify the flow generated by the ventricular chamber. The integrated microfluidic system recapitulates the ventricular fluidic function and exhibits a complete pressure-volume loop with isovolumetric phases. Together, our results demonstrate a previously unexplored application of high-precision fabrication that can be generalized to expand the accessible spectrum of organ-on-a-chip models toward structurally and biomechanically sophisticated tissue systems.
Biomimetic tissue models are rapidly evolving toward elaborate, next-generation in vitro culture models to study organ-level function during development, adulthood, regeneration, and disease . The development of such models can be attributed to progress in induced pluripotent stem cell (iPSC) technology, as well as innovative methods to assemble cells into aggregates and functional structures, from organoids to different types of three-dimensional organ-on-chip models. In recent years, staggering advances have been made in differentiating stem cells into a variety of lineages and in triggering them to do so in multicellular organoids, largely built on insights from recapitulating developmental signals. Despite these advances, the lack of approaches to generate higher-order structural and anatomical features at the micrometer scale has made it difficult to model organ-level functions in vitro. For instance, while cardiac, skeletal, and smooth muscle cells have been derived from iPSCs, attempts to organize these cells to recapitulate the many different muscular architectures that give rise to different biomechanical functions have been sparse.
The ability to build more complex structures has been hindered partly by the poor adaptation of fabrication approaches with the nanometer-to-millimeter fabrication length scales relevant to tissue engineering. Here, we explore the use of two-photon direct laser writing (TPDLW), a high-resolution fabrication method, to build biological systems requiring specific architectures and functions. As a challenge, we engineered a microfluidic cardiac chamber powered by human iPSC-derived cardiomyocytes (hiPSC-CM), aiming to replicate the ventricular function on a chip. While current state-of-the-art cardiac tissue models are well suited to measure contractile forces generated by cardiomyocytes, modeling the cardiac pumping function using engineered cardiac tissues has been challenging. Here, we harness the high precision of TPDLW to implement a miniaturized metamaterial scaffold that supports the anisotropic contraction of an in vitro cardiac ventricle, as well as functional miniaturized valves featuring sufficient sensitivity to respond to the cardiac chamber’s flow. The integration of the chamber and valves within a microfluidic device recapitulates the unidirectional volumetric output of the human ventricle and exhibits a complete ventricular pressure-volume (PV) loop. Our cardiac miniaturized precision-enabled unidirectional microfluidic pump (miniPUMP) system sets a precedent for a general approach to the assembly of sophisticated tissue structures that leverages the benefits of high-precision manufacturing to recapitulate whole-organ function
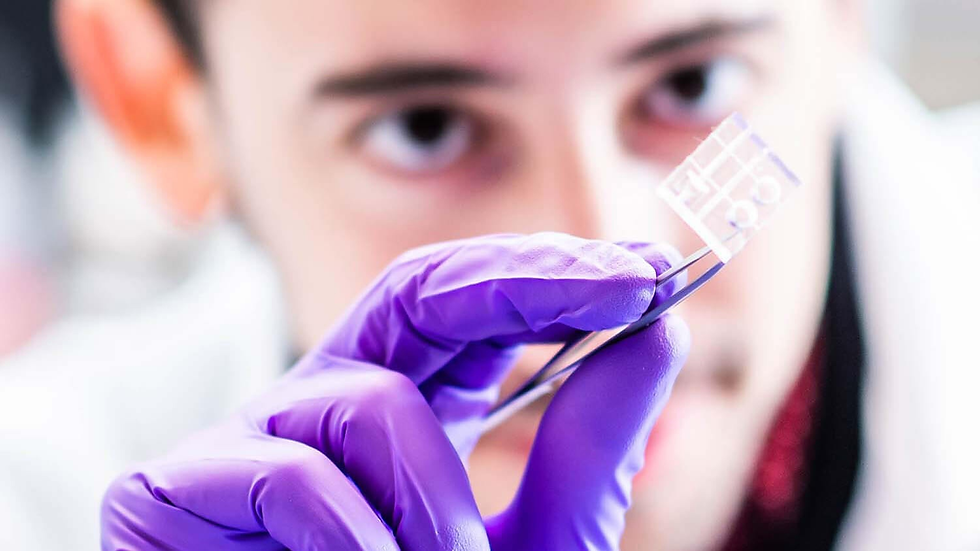
To emulate cardiac output, we envisioned a microfluidic system that would replicate the main features of the human ventricle, namely, a contracting myocardial chamber that generates flow, cardiac valves that rectify the generated flow, and a pressure gradient between the venous blood supply and the arterial output vessel of said flow. We first set out to build a microscopic, hollow, cylindrical scaffold (approximately 1-mm inner diameter and 1-mm height) that would guide the assembly of hiPSC-CMs in a chamber format within the miniPUMP device. To mimic the auxetic compression of the cardiac chambers for maximum ejection volume, we first fabricated chamber scaffolds composed of auxetic inverted hexagon unit cells using TPDLW to achieve submicrometer resolution of the individual beams. However, structural instabilities ultimately caused asymmetric collapse of the structures under contractile forces generated by seeded hiPSC-CMs. To address this instability, we restricted chamber deformability to the axial direction using an improved scaffold design. The improved design consisted of two sets of fine flexible concentric helices that were connected to each other in the transverse plane through thick rigid horizontal rings. The rings were narrowly spaced in the axial direction to prevent radial constrictions of the scaffold that could compromise its stability. Importantly, compressive mechanical tests indicated that the axial stiffness of the helical scaffolds could be repeatably controlled by varying the helix cross-sectional thickness, for example, increasing from 41.7 ± 1.9 to 71.3 ± 1.5 N/m when the thickness increased from 8.3 ± 0.2 to 10.1 ± 0.3 μm. Therefore, cardiac chambers based on this helical scaffold allowed us to rapidly and empirically identify a helix design that could sustain substantial tissue contractions and allowed generation of flow without collapsing over the span of a week . Although the scaffold exhibited a largely linear stiffness with slight strain softening that does not mimic the strain stiffening of native contracting myocardium, the hiPSC-CMs remained viable, spread on the scaffolds, and formed thick multilayered tissues with dense sarcomere networks, suggesting that the scaffold did not exhibit major cytotoxic effects.
Source: Science.org
Comentários